(The Facts About Lighting and Vision)
by Dan Roberts, Founding Director Macular Degeneration Support
Originally published on MDSupport, updated October 3, 2011)
Introduction
What Is Light?
The Blue Light Hazard
Terms Referring to Measurement of Light
Types of Lamps
The Problem With Full-Spectrum Lamps
Market Survey
Summary
Discussion
Safe Options
Live Comparison of Representative Lamps
A Message To The Industry
Random Quotes
Credits
References
Introduction
Research continues to warn about the danger of sunlight to the eyes. Doctors wisely respond by recommending eye protection while outdoors during daylight. Curiously, however, some lighting manufacturers say lamps duplicating sunshine are good for vision and eye health. This presents a quandary that needs to be addressed.
Who is right? How does light hurt the retinas? What are the differences between fluorescent, halogen, neodymium, LED, and regular incandescent lightbulbs? What is meant by “full spectrum” and “daylight?” What kind of lighting is best and safest? Before approaching these questions, it is important to have a basic understanding of light and its effects on the retina.
Light is made up of electromagnetic particles that travel in waves. The human eye responds to only a small part of the entire electromagnetic spectrum. From the longest waves (lowest frequency) through the shortest waves (highest frequency), lighting specialists identify the electromagnetic wave regions as 1) radio waves, 2) microwaves and radar, 3) millimeter waves and telemetry, 4) infrared, 5) visible light, 6) ultraviolet, and 7) x-rays and gamma rays.
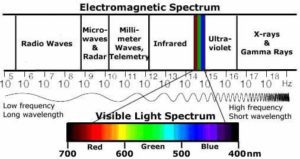
(Fig. 1)
The retina is a very thin, multi-layered tissue located at the back of the eyeball. The lens at the front of the eyeball focuses light onto it.
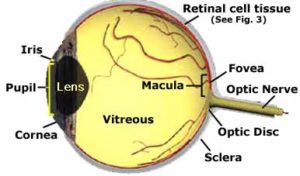
The light is received by photoreceptor cells called rods (responsible for peripheral and dim light vision) and cones (providing central, bright light, fine detail, and color vision). The photoreceptors convert light into nerve impulses, which are then processed by the retina and sent through nerve fibers to the brain.
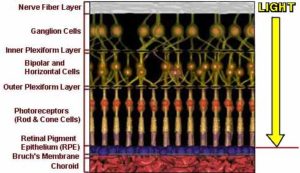
(Fig. 3)
The Blue Light Hazard
Sight requires light. As years go by, accumulation of lipofuscin (cellular debris) in the retinal pigment epithelium (RPE) may make the retina more sensitive to damage from chronic light exposure.6 7 8 9 10 11 12 13 14 15 Retinal light damage has been studied by exposing experimental animals and cell cultures to brilliant light exposures for minutes to hours. According to some of these studies,16 17 18 19 blue light waves may be especially toxic to those who are prone to macular problems due to genetics, nutrition, environment, health habits, and aging. On the other hand, acute retinal phototoxicity experiments such as these can cause retinal injuries, but they cannot simulate a lifetime of normal light exposure. Some researchers have noted strong similarities between photic injury and retinal abnormalities caused by years of overexposure to light.47 48 49 50 Others have found no similarities. 51 52 53 54 55 56 57 58 Whereas the shorter wavelengths of UV-A and UV-B are somewhat filtered by the lens and cornea, animal studies have shown that the light spectrum from UV through blue can be harmful. During lengthy exposures of up to 12 hours, toxicity of the retina is known to increase as the light wavelengths grow shorter.20 21 22 23 24 25 26 27 28 29 30 31 32 33 56 More recently, research on human fetal cell tissue has also revealed damage from blue light exposure.78 Fortunately, healthy retinas have a wide array of built-in chemical defenses against UV-blue light damage. They bear such imposing names as xanthophyll, melanin, superoxide dismutase, catalase, and glutathione peroxidase. And then there are the more familiar agents vitamin E, vitamin C, lutein, and zeaxanthin. 35 36 37 38 39 Unfortunately, these defenses can weaken with disease, injury, neglect, and age.Another built-in protective process is that the natural lens takes on a yellowish tint with age, which helps to filter blue light.59 60 After cataract surgery, however, patients lose that benefit. Some doctors now recommend replacing the damaged lens with an intraocular lens (IOL) that is tinted to block blue light.79 The patient should be made aware, however, that this procedure will diminish scotopic (night) vision.61 62
According to the CVRL Color & Vision database,63 light waves measuring approximately 470nm to 400nm in length are seen as the color blue. The blue bands of the visible light spectrum are adjacent to the invisible band of ultraviolet (UV) light. UV is located on the short wave, high frequency end of the visible light spectrum, just out of sight past the color violet. It is divided into three wavelengths called UV-A , UV-B, and UV-C. The effects of UV-C (100nm-290nm) are negligible, as the waves are so short they are filtered by the atmosphere before reaching the eyes. UV-A (320nm-400nm) and UV-B (290nm-320nm) are responsible for damaging material, skin, and eyes, with UV-B getting most of the blame.
When light hits a photoreceptor, the cell bleaches and becomes useless until it has recovered through a metabolic process called the “visual cycle.” 30 31 Absorption of blue light, however, has been shown to cause a reversal of the process in rodent models. The cell becomes unbleached and responsive again to light before it is ready. This greatly increases the potential for oxidative damage, which leads to a buildup of lipofuscin in the retinal pigment epithelium (RPE) layer64 (see Fig. 3). Drusen are then formed from excessive amounts of lipofuscin, hindering the RPE in its ability to provide nutrients to the photoreceptors, which then wither and die. In addition, if the lipofuscin absorbs blue light in high quantities, it becomes phototoxic, which can lead to oxidative damage to the RPE and further cell death (apoptosis).65
Blue light is an important element in “natural” lighting, and it may also contribute to psychological health.71 72 Research, however, shows that high illumination levels of blue light can be toxic to cellular structures, test animals, and human fetal retinas.56 66 67 68 69 70 78 79 80 81 (Also see “Random Quotes” below.) The industry has established standards for protecting consumers from extremely bright light and from UV radiation; but no standards address the blue light hazard that may be affecting millions who have retinal problems. Blue light is a duplicitous character who needs to be carefully watched. Until research proves him to be either a friend or a foe, education will help consumers make decisions based upon the facts.
The next section defines the terms used in the lighting industry. Knowing the language will help a great deal with understanding.
Terms Referring to Measurement of Light
Color Rendering Index (CRI):
The CRI of a lamp is a number from 20 (effectively) to 100 that describes how well the lamp’s light emission affects the appearance and vibrancy of an object’s color. It is determined by comparing the lamp with a reference source of the same Kelvin temperature (see below).
Kelvin (K):
Kelvin is the basic unit of measurement for temperature. 0 Kelvin = -273.15° centigrade. The Kelvin temperature rating is based on the color most highly emitted. It does not express the range of a lamp’s light spectrum or the strength of it’s illumination (radiant power).
Correlated Color Temperature (CCT):
The CCT number is a measurement of the actual color appearance of light. It is expressed in Kelvins. Low CCT numbers define “warm” lighting, like the yellow and red hues of candlelight at 1500K. High CCT numbers define “cool” lighting, like a clear blue sky at 5000K. Actual visible light measures from a low of 800K, below which it begins to enter the invisible infrared range, to a high of 12,000K, above which it begins to enter the invisible ultraviolet range.
Footcandle (fc) and LUX (lx):
FC and LX are unit’s of illuminance(light on a surface). 1fc=lm/ft2. 1lx=lm/m2. 1lx=0.0929fc. 50 footcandle is generally considered sufficient for most tasks.
Lumen (lm):
A lumen is the standard unit of luminous flux (the time rate of flow of radiant energy). This is a measurement at the light source (the lamp), not necessarily at the surface being lit.
Nanometer (nm):
A nanometer is the extremely small unit used to measure lengths of light waves. A single nm equals one billionth of a meter.
Watt:
A watt is a unit of power equal to work done at the rate of one joule (approximately 0.738 foot pounds) per second. Wattage is actually a measurement of energy, not of light.
Types of Lamps
What is a lamp?
Contrary to popular usage, a lamp is neither a fixture that holds a lightbulb or tube, nor is it “a light.” A lamp is the lightbulb or tube itself, which is contained in the lighting “fixture” (or “instrument”). Light is the energy that emits from the lamp. Only incandescent lamps, by the way, should rightfully be called lightbulbs, due to their bulbous (i.e. fat and round) shape.
Full-Spectrum (FS):
Technically, there is no such thing as a true full spectrum lamp, but the term is used to define a light source with a CCT of 5000K or higher and a CRI of 90 or higher. FS lamps are fluorescent, and they often have enhanced levels of UV. Only lamps that meet these specifications should be called FS.
Fluorescent:
This type of lamp is a phosphor-coated tube filled with mercury and argon vapor. Phosphors in lamps are rare earth compounds of various types that glow during absorption of light radiation. An electrical current discharged into the vapor causes the phosphor to glow (fluoresce). Fluorescent lamps require an electrical component (a “ballast”) to create the arc that excites the gas. The type and blend of phosphors used in the coating determine the color of the emitted light.
Fluorescent tubes containing the older halophosphate type phosphors emit light that is high in the blue spectrum. The phosphors increase the wavelength of the invisible UV rays enough to convert them into visible light beginning at 400nm. In common fluorescent tubes, UV rays are blocked mostly by the glass enclosure, protecting the eye to some extent from those harmful wavelengths. The blue light, however, passes through unimpeded.
Most fluorescent tubes now use a triphosphor mixture, based on europium and terbium ions, which more evenly distributes over the visible light spectrum. With a blend of phosphors designed for a CCT of 5000K-6500K, these lamps come close to imitating the colors of daylight. Blue light is an important component of that mixture, so the 470nm-400nm band is not only unfiltered, it is often enhanced in the manufacturing.
Incandescent:
Commonly known as a lightbulb, an incandescent lamp contains a tungsten filament in a vacuum. An electrical current causes the filament to glow (incandesce), while the absence of oxygen keeps it from burning up.
Neodymium:
This is a natural heavy metal element used as a coating on the inside of some light bulbs. It filters out the yellow spectrum, thus creating a CRI closer to that of daylight. Neodymium bulbs, therefore, are often marketed as one of the types of modified-spectrum lamps, but they should not be confused with FS lamps.
Tungsten Halogen:
Also called halogen, this lamp contains a filament made of tungsten, so it is a type of incandescent lamp. It is different than an incandescent lightbulb, however, in that it contains a gas called halogen. Halogen recycles the burned particles of the tungsten, constantly rebuilding the filament and giving it a longer life. Halogen burns very hot and bright, so it can be a safety hazard if not properly used.
Light Emitting Diodes (LEDs):
An LED is basically a semiconductor diode. When the diode is switched on, electrons are able to recombine with electron holes, releasing energy in the form of light. The LED is usually less than 1 mm squared in area and contains components to shape its reflection and radiation pattern. LEDs are economical, efficient, durable and small. They are used in home, theater and automotive lighting, traffic signals, text and video displays, and communications technology, to name only a few of the growing number of applications.
The amount of harm from an LED, like any other lamp, is determined by the built-in protection of the product, in combination with reasonable precautions taken by the viewer. Specific to LEDs, the wider the field of light (i.e. size of the illumination source) and the brighter (higher luminance) of that source, the more potential risk it carries for the retina. Proximity to the light source is also a consideration. One should not, for example, gaze up close into a light box of blue (or even white) LEDs for longer than 100 seconds (the maximum recommendation from the International Commission on Non-Ionizing Radiation Protection). On the other hand, a small array of white LEDs (which contain some of the blue spectrum) in a lamp used for task lighting would probably cause little problem.
The Problem With Full-Spectrum Lamps
With growing evidence that both UV and blue light damage the retinas of people affected by, and at risk of, retinal disease, everything possible needs to be done to avoid aggravating the condition. Consumers should arm themselves with good information to protect against the advice of marketers who may not be familiar with (or who ignore) the possible hazards.
Manufacturers promote FS lamps as more conducive to seeing than traditional lighting systems, based upon the belief that people see best outdoors on a bright cloudless day. That assumption is disputed by others who suggest that better vision under such conditions may be a result of increased light intensity and uniformity rather than color. No known studies that have yet tested this hypothesis. Part of the reason for that paucity may be because FS lamps are difficult to define and compare. The essence of daylight is constantly changing as Earth moves in relation to the sun, and varied atmospheric conditions throughout the world measure differently.73 In view of such variables, “daylight” is a vagarious term with no standard scientific definition, so it really has no meaning in the lighting market.
The eye inarguably sees better in the light of day than in the dark of night. A good deal of research is showing, however, that the light of day (i.e. high in the UV-blue spectrum) may be deleterious to the retinas. Since light is both practical and desirable, it is necessary. At the same time, people with retinal dystrophies need to diminish the progression of vision loss. Until good science provides more definite answers, therefore, it might be wise to not bring the sun into the house and place it on the desktop.
Market Survey
In an attempt to learn more about such products currently on the market, information was gathered on 19 “full spectrum” or “daylight” lamps offered by 12 companies during the year 2004. Conclusions were then drawn from their published CCTs, CRIs, and descriptions.
Survey of Lamps Labeled as “Full Spectrum” or “Daylight”
On the Market as of December 31, 2004
- CCT: 6500K
- CRI: 82-84
- Type: Neodymium
- Described as contributing to vision health.
UPDATE: No longer claims to contribute to vision health.
[Technically not full spectrum, but CCT surpasses recommended minimum.]
BioPure Full Spectrum Light Bulb (marketed by Joseph Mercola, D.O.)
- CCT: 5500K
- CRI: 93
- Type: Fluorescent
- Described as “full spectrum.”
- Advertising recommends not filtering any of the visible or UV spectrum: “Unfiltered sunshine is important. If you are wearing glasses or sitting in front of a window, some of the 1500 wavelengths present in sunshine will not reach your retina and nourish your brain.”
UPDATE: Appears to be discontinued
“Bright As Day!” Desk Lamp (Sharper Image Design)
- CCT: 5000K
- CRI: 91
- Type: Fluorescent
- Described as “wide spectrum” and “daylight spectrum.”
UPDATE: Appears to be discontinued
Chromalux by True Sun
- CCT: 5000K
- CRI: 50
- Type: Neodymium
- Described as contributing to vision health.
- Advertising implies that it is a “full spectrum” lamp. Quote: “…provides bright light that closely mimics the spectrum of Natural Sunlight (infrared, visible spectrum and beneficial U.V.A. rays).”
UPDATE: Now advertises:
- High color temperature (4200K and 4700K) for enhanced whiteness
- No UV (ultraviolet light) emission
[No longer considered full spectrum]
Coil-Lite Compact Fluorescent by True Sun
- CCT: 6500K
- CRI: 82
- Type: Fluorescent
- Described as “full spectrum” lamp.
UPDATE: Appears to be discontinued
Full Spectrum (VisionMax) by Tensor
- CCT: 6500K
- CRI: 84
- Type: Fluorescent
- Described as “full spectrum” lamp.
- Advertising quotes: “True-to-life colors.” “Vivid contrast.” “The ultimate natural daylight lamp.” “Replicates sunshine indoors.” “Especially for aging eyes.”
UPDATE: No longer claims “Especially for aging eyes.”
[Technically not full spectrum, but CCT surpasses maximum]
Life-Lite by True Sun (replaces Vita-Lite by Duro-Test Light Corp.)
- CCT: 5500K
- CRI: 91
- Type: Fluorescent
- Described as “full spectrum” lamp.
UPDATE: Appears to be discontinued
Lumichrome 1XX by True Sun
- CCT: 6500K
- CRI: 98
- Type: Fluorescent
- Described as “full spectrum” lamp.
- Advertising quote: “…replicates the characteristics of diffused natural sunlight.”
Neolite light bulbs by Full Spectrum Solutions
- CCT: 4800K
- CRI: 95
- Type: Neodymium
- Described as “full spectrum” lamp (contrary to standard definition).
- Advertising quote: “…similar to true daylight.”
[Technically not full spectrum, since CCT is below 5000K. Considered safe for low vision users.]
Ott-Lite (Developed by Vita-Lite)
- CCT: 5000K
- CRI: 79-82
- Type: Fluorescent
- Described as “full spectrum” lamp.
- Described as contributing to vision health.
- Advertising quote: “the next best thing to natural daylight.”
UPDATE: No longer claims to “contribute to vision health”, but instead includes a doctor’s testimonial: “…it’s the only light that I recommend to my patients.”
[Technically not full spectrum, but CCT meets maximum. CRI is too low to offer best contrast.]
Paralite-Maxum 5000 by Full Spectrum Solutions & True Sun
- CCT: 5000K
- CRI: 88-91
- Type: Fluorescent
- Described as “full spectrum” lamp.
Paralite-Spectra 5900 by Full Spectrum Solutions & True Sun
- CCT: 5900K
- CRI: 93
- Type: Fluorescent
- Described as “full spectrum” lamp.
PureLite by Natural Lighting
- CCT: 5000K
- CRI: 90
- Type: Neodymium
Phillips TL 90 Series-T8 Fluorescent Lamps by Full Spectrum Solutions & True Sun
- CCT: 5000K
- CRI: 98
- Type: Fluorescent
- Described as “full spectrum” lamp.
Sunlight Lamp by Bell & Howell
- CCT: 6500K
- CRI: 80-82
- Type: Fluorescent
- Description as “full spectrum” or “daylight” lamp is implied by its name.
[Technically not full spectrum, but CCT surpasses maximum]
UltraLux by Full Spectrum Solutions
- CCT: 5500K
- CRI: 91+
- Type: Fluorescent
- Described as “full spectrum” lamp. Doubles as therapy lamp by tilting to shine directly on the user.
Verilux Happy Eyes
- CCT: 5500K
- CRI: 80-82
- Type: Fluorescent
- Description as “full spectrum” and “daylight” lamp implied in advertising: “natural spectrum,” “simulating daylight,” and “sunshine in a box.”
- Described as beneficial to patients with computer vision syndrome.
[Technically not full spectrum, but CCT surpasses maximum]
Vita-Lite by Natural Lighting
- CCT: 5000K
- CRI: 79
- Type: Fluorescent
- Described as simulating full color and UV spectrum.
[Technically not full spectrum, but CCT meets maximum]
Vita-Lite Plus by Natural Lighting
- CCT: 5500K
- CRI: 91
- Type: Fluorescent
- Described as “full spectrum” lamp
- Advertising quote: “Enhanced with UV and blue-green phosphors”
UPDATE: Advertising quote has been removed.
Summary
(Updated January 20, 2018)
- Number of lamps surveyed=19
- Lamps defined as fluorescent=15
- Lamps defined as neodymium=4
- Lamps correctly described as “full spectrum”=9
- Lamps incorrectly described/implied as “full spectrum”=7
- No claims made regarding “full spectrum” or “daylight”=2
- Lamps advertised as having enhanced or non-filtered UV (orig. 2)=1
- Lamps advertised as healthy for the eyes (originally 7)=4
- Lamps discontinued=3
A commercial market will inevitably grow around any human condition with profit potential. For the best protection of health and pocketbooks, consumers should maintain vigilance about the facts and skepticism about anything that doesn’t pass the test of reason. At first hearing, for example, “Bright daylight is good for the eyes,” seems to make sense. But if that is so, then . . .
- Why do the eye’s pupils constrict in sunlight?
- Why do skiers go snow blind?
- Why shouldn’t a person look at the sun?
- Why do eye doctors recommend wearing wraparound, UV-protective, blue-blocker sunglasses?
Seven of the surveyed companies actually advertised their FS lamps as good for eye health. Common sense should suggest otherwise, but common sense can sometimes be overridden even by people with the best of intentions.
Grandmother warned, “Turn on the light. You’re going to ruin your eyes. Reading in the dark makes them work too hard.” She meant well, but her suggestion should have been simply, “Turn on the light. You’ll be able to see better.”
Eyes don’t work any harder at seeing in the dark than do ears at hearing soft music. Actually, the demand on the photoreceptor cells increases as the light grows brighter. This may prove harmful to the vision of people with retinal problems, so it might be smart to reach a compromise between “enough light to see by” and “too much light.”
The condition of individual retinas determines the level of light required. A person with early-stage macular degeneration can usually read well enough under standard lighting. A person with advanced-stage macular degeneration, however, requires higher illumination and contrast. Ironically, many are also photosensitive. They need bright lighting, but lamps that replicate sunshine might not only hasten sight loss, they can cause significant discomfort. “Enough light to see by” cannot be standardized.
A growing body of research shows that the shorter electromagnetic waves of the light spectrum from blue (also called near-UV) through ultraviolet may be harmful. One study even suggests that lower color temperature (i.e. reduced blue light) optimizes the reading rate of people whose visual abilities have been lessened due to ARMD.74 A study of the Chesapeake Bay watermen reported a significant association between blue light and age-related macular degeneration.75 76 In view of this kind of scientifically-based evidence (no matter how small or large the studies might be), people who are at risk for retinal degeneration might be wise to consider avoiding light sources measuring higher than 5000K at high intensity.
Lamps that imitate daylight and sunlight are said to help people see better, and some are even credited with helping to improve mood. They will, however, neither improve the health nor extend the life of retinal cells, a scientifically unsupported implication made by some manufacturers. Such lamps may be helpful for people with healthy eyes, but those who aren’t so lucky should think twice. Major research shows that sunlight is a possible causative element in several retinal diseases, from retinopathy of prematurity to age-related macular degeneration.77 19. That kind of evidence should be enough to make those who are already visually-impaired wary of FS lamps.
At the time of the survey, one distributor had established an important precedent by publicly recognizing potential optical risks associated with high levels of blue light. The company is Sunnex Biotechnologies, makers of the Lo-Light Therapy Lamp (a safe low-intensity lamp that screens out blue light). Here is a cautionary statement based upon information from their web site. It might serve as a model for makers of FS lamps to follow:
CAUTION: This lamp emits strong blue light wavelengths (470nm – 400nm), which have been shown in some studies to harm the retina. Avoid prolonged use of this product if . . .
|
Several lamp manufacturers provide products that offer intensity, contrast, and good color replication without the high color temperatures of full spectrum lamps. Listed below are examples of bright desk lamps and task lamps on the market with CCTs below 5000K. So far, these and similar products represent the safest for low vision people.
Allievo, Elegance, and Junior LED Lamps (www.berryessadesigns.com)
- CCT: 2600K ~ 3700K
- CRI: >80
- Type: Light emitting diode (LED)
- “Designed with the needs and safety of the low vision community in mind.”
- Described as “the most economical high brightness lamp.”
- Described as “soft warm-white light.”
LazLight Task Lamp (no longer available)
- CCT: 3000K
- CRI: 99
- Type: Halogen with dimmer
- Described as “high performance white light.”
- Described as “brightest-available high-contrast true-color task lamp.”
- Described as “350% more light . . . with no damaging blue light radiation.”
SoLux Task Lamp and Solux Touch-On Dimmable Lamp (www.solux.net)
- CCT: 3500K – 4700K
- CRI: 98-99
- Type: Halogen and halogen with dimmer
- Described as “daylight” lamp.
- Described as “closest to true spectrum” with no UV or IR radiation.
Live Comparison of Representative Lamps
To demonstrate the differences in lamp types, lighting products mentioned in this paper were acquired and compared. The demonstration was designed with six lamps for an exhibit at the 2005 meeting of the Academy for Research in Vision and Ophthalmology (ARVO) and at other gatherings of researchers, eye care professionals, and patients. Since then, an LED lamp has been added to the display. This section describes the display and discusses the most typical observations made by viewers.
Task lamps compared:
1. General Electric Basic 75w, 2700K incandescent
2. LazLight 3000K incandescent halogen
3. Solux 4100K incandescent halogen
4. RobinSpring32 3200K compact fluorescent
5. Ott-Lite 5000K compact fluorescent
6. Full Spectrum (VisionMax) 6500K compact fluorescent
7. Allievo (Berryessa Designs) 2600K ~ 3400K white LED
Set-up:
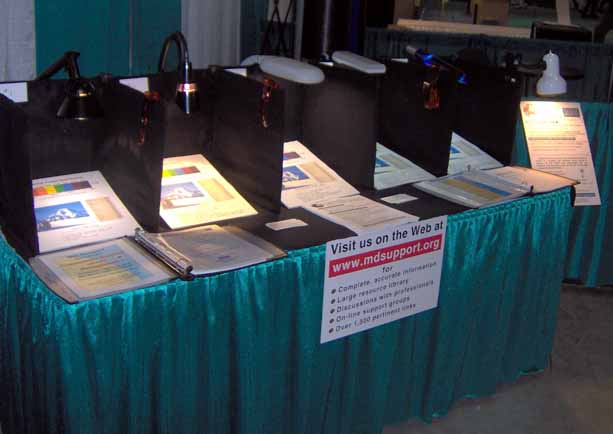
MD Support display at the 2005 convention of the Association for
Research in Vision and Ophthalmology (ARVO)
The lamps are each placed to shine into individual sections of a 6-foot long display unit. All lamp heads are equidistant from the surface and shining at maximum power. They each illuminate posters that display the following content:
- Identical photos of a predominantly blue mountain scene.
- Actual pages from a typical telephone directory.
- A graphic of each lamp’s spectral distribution curve of radiant power overlaid on an image of the visible color spectrum.
- Name and description of each lamp, including the CCT, CRI, type, and retail cost.
Sample poster:
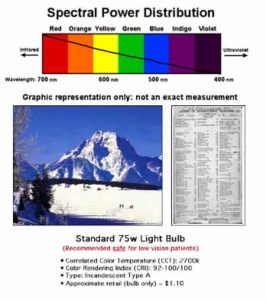
The viewer is instructed to:
- Observe each lighted section with the naked eye and then through an amber-colored gelatin sheet or 100% blue-blocker lenses.
- Note the differences in terms of light intensity, contrast, color, and general effect.
- Compare each illuminated area to its own spectral distribution curve.
Typical Observations and Discussion
“Some lamps are bluer.”
Blue light emission becomes more apparent as correlated color temperature (CCT) increases for each type of lamp. The blue light intensities of the lamps can be compared by viewing the posters through the amber gel provided or through 100% blue-blocker lenses. Where the CCT is low (lamps 1,2 and 7), blue appears gray and black. As the CCT increases from lamps 3 through 6, the blue portions appear increasingly brighter as the color green.
Viewing with the naked eye the same picture lit with lamps of different color temperatures and keeping all other conditions equal, one notices distinct differences in hue. As is evident in the photographs below, worst contrast (i.e. highest blue content) is provided by fluorescent lamps with ≥3000K color temperatures. Poor color replication also occurs with any lamp with a color temperature of ≤3000. Best contrast (i.e. whitest whites and blackest blacks) and best color replication in this comparison is provided by the 2600K ~ 3400K white LED light source.
![]() 2700K incandescent light bulb |
![]() 2700K spiral fluorescent |
![]() 3000K compact fluorescent |
![]() 5000K compact fluorescent |
![]() 3000K halogen |
![]() 3600K white LED |
“Some lamps are brighter.”
When all are at full power, the fluorescent lamps 4-6 are less intense than the incandescent and LED lamps. The high amount of blue in the light of lamps 4-6 deceives the brain into interpreting them as “bright” until they are observed side by side with the others.
Incandescent and LED lamps are capable of several times the intensity of fluorescent lamps, and there is little danger of reaching hazardous blue light levels. If the intensities of the fluorescent lamps were increased to match the obviously stronger output of the others, the amount of blue light would also increase, possibly compounding any hazard to the retina. The only way to safely increase the intensities of fluorescent lamps to match that of incandescent and halogen lamps would be to significantly decrease the blue and UV wavelengths. Such lamps, however, could then no longer be called full-spectrum or daylight.
“Some of the lamps are too bright for me.”
Some incandescent and LED lamps have dimmers, which allow users to adjust the intensity to various comfort levels. Fluorescent lamps normally do not have dimmers, but they can be found.
“Some lamps provide more contrast.”
Contrast diminishes with increasing CCT. Because of their “blueness,” lamps 5-6 provide noticeably less contrast than lamps 1-4 and 7. The best contrast is achieved by the opposites of black and white, and that cannot be done with blue light.
“Some lamps are too intense for my eyes.”
Lamps 5-6 are not as easy on the eyes as lamps 1-4 and 7. Because of its “blueness,” a cloudless day at noon (i.e. “daylight” at 5000K) is not as comfortable for the eyes as the warmer colors of late afternoon. Lamps 1-3 emit light closer to the warm yellow and orange end of the visible color spectrum, with lamps 3 and 7 being the “whitest,” due to the relative evenness of their outputs across the visible spectrum.
“The safest lamp seems to be a standard incandescent light bulb. So why spend more for halogen, warm white fluorescent, or white LED lamps?”
Standard incandescent light bulbs (eg. Phillips Type A) cost more to operate, and they have significantly shorter life spans. Also, they emit yellowish light, whereas whiter light is preferred for better contrast and color replication by professional crafters, photographers, and artists. People with low vision from retinal disease need white light for the same reason.
“Why is there such a difference in the prices?”
The cost of the lamps themselves (the light bulbs, tubes, and LEDs) is similar for like-models. The quality of the fixtures holding them actually determines the bulk of the cost. Some fixtures are plastic, some are steel. Some have dimmers, some don’t. Some are designed to complement a decor, some are merely functional. Some are made to last, some are not.
“Halogen, standard incandescent, warm-white fluorescent, and white LED lamps provide better lighting than full spectrum lamps.”
This is the opinion of virtually every viewer who has compared the lamps side-by-side. 100% of more than 150 doctors surveyed at the ARVO convention concurred that the display lamps measuring below 5000K provided the best illumination, contrast, and color replication. Their conclusion was reached notwithstanding the blue light issue. Even those who do not fully agree with the research about the blue light hazard agree that low vision patients should be guided away from full spectrum lamps, if only because of their comparatively poor quality of light emission.
A Message To The Industry
The message from the low-vision community is simple:
- Include a warning if the product presents a potential blue light hazard for people with retinal disease.
- Provide accurate and complete specifications and descriptions so the consumer can make educated comparisons.
In view of the expanding volume of research, plus the increasing number of people diagnosed with retinal disease each year, manufacturers are encouraged to voluntarily join the low vision community and eye care specialists in this education effort. Modern lighting technology can help people see better, but only through clear and honest marketing will the liability for proper decisions be theirs alone.
Cautionary Statements From The Literature
” …people with the highest levels of exposure [to UV-A, UV-B, and blue light] in the middle of the day had two fold increased risks of AMD. Our results showing the increased risk from high exposures to solar radiation underline the importance of ocular protection in European populations.” Augood, C. et al. Age-related maculopathy and macular degeneration in elderly European populations: the EUREYE study, 2004.
“The photoreceptors in the retina . . . are susceptible to damage by light, particularly blue light. The damage can lead to cell death and diseases.” Shaban H, Richter C. A2E and blue light in the retina: the paradigm of age-related macular degeneration. Biol Chem 2002 Mar-Apr;383(3-4):537-45.
The effectiveness of light in inducing photodamage to the retina increases with decreasing wavelength from 500 to 400 nm.” Andley UP,? L.T. Chylack Jr LT. Recent Studies on Photodamage to the Eye with Special Reference to Clinical and Therapeutic Procedures. Photodermatology Photoimmunology and Photomedicine 1990; 7:98-105.
“. . . when albino rats were exposed to either monochromatic blue light of 403 nm . . . or monochromatic green light of 550 nm . . . massive apoptotic cell death occurred after illumination with blue light.” Remé et al. Apoptosis in the Retina: The Silent Death of Vision C E. News Physiol Sci 15: 120-124, 2000.
“. . . continuous exposure to blue light is potentially dangerous to vision.” Koide R, Ueda TN, Dawson WW, Hope GM, Ellis A, Somuelson D, Ueda T, Iwabuchi S, Fukuda S, Matsuishi M, Yasuhara H, Ozawa T, Armstrong D. Nippon. Retinal hazard from blue light emitting diode. Ganka Gakkai Zasshi. 2001 Oct;105(10):687-95.
“. . . high levels of exposure to blue or visible light may cause ocular damage, especially later in life, and may be related to the development of age-related macular degeneration.” Taylor HR, West S, Munoz B, Rosenthal FS, Bressler SB, and Bressler NM. The Long Term Effects of Visible Light on the Eye. Archives of Ophthalmology 1992; 110:99-104.
“I think chronic blue light is probably damaging.” Joshua Dunaief, MD, in Bethke W. Should We Block The Blue. Review of Ophthalmology Oct 15 2003; 10(10).
“Increased risk of AMD may result from low levels of lutein and zeaxanthin (macular pigment) in the diet, serum or retina, and excessive exposure to blue light.” Bone RA, Landrum JT, Guerra LH, Ruiz CA. Lutein and Zeaxanthin Dietary Supplements Raise Macular Pigment Density and Serum Concentrations of these Carotenoids in Humans. Journal of Nutrition 2003 Apr;133(4):992-8.
“The high-energy segment of the visible region (400-500 nm) is enormously more hazardous than the low energy portion (from 500-700 nm).” Young RW. Solar Radiation and Age Related Macular Degeneration. Survey of Ophthalmology 1988; 32(4): 252-269.
“Visible light of short wavelength (blue light) may cause a photochemical injury to the retina, called photoretinitis or blue-light hazard.” Okuno T, Saito H, Ojima Evaluation of blue-light hazards from various light sources. J. Dev Ophthalmol. 2002;35:104-12.
“[The] Action spectrum for blue-light induced [retinal] damage shows a maximum at 400 nm and 450 nm.” Bartlett H, Eperjesi F. A randomised controlled trial investigating the effect of nutritional supplementation on visual function in normal, and age-related macular disease affected eyes: design and methodology. Nutrition Journal 2003, 2:12.
“Because sunlight and many high-intensity artificial light sources contain relatively high proportions of blue, and the retina as well as pigment epithelium contain several types of blue-absorbing molecules, the short-wavelength band of the visible spectrum may contribute to the pathogenesis of age-related macular degeneration and amplify some forms of inherited retinal degeneration.” Remé CE, Wenzel A, Grimm G, Iseli HP. Mechanisms of Blue Light-Induced Retinal Degeneration and the Potential Relevance for Age-Related Macular Degeneration and Inherited Retinal Diseases SLTBR Annual Meetings Abstracts 2003.
“. . . the photon catch capacity of the retina is significantly augmented during blue-light illumination, which may explain the greater susceptibility of the retina to blue light than to green light. However, blue light can also affect function of several blue-light-absorbing enzymes that may lead to the induction of retinal damage.” Grimm C, et al. Rhodopsin-Mediated Blue-Light Damage to the rat Retina: Effect of Photoreversal of Bleaching. Invest Ophthalmol Vis Sci 2001 Feb;42(2):497-505.
“It is not too harsh to state that virtually all persons with vision problems should be removed from a light environment where the predominant light waves are a temperature above 3500K or a wavelength less than approximately 500 nm.” Elaine Kitchel, M.ED.VI. The effects of fluorescent light on the ocular health of persons with pre-existing eye pathologies. American Printing House for the Blind, 2000.
“Exposure to the eye to intense light, particularly blue light, can cause irreversible, oxygen-dependent damage to the retina. We have found that illumination of human retinal pigment epithelium cells induces significant uptake of oxygen that is both wavelength and age dependent…and contribute to the development of age-related maculopathy.” Rozanowska M, et al. Blue light induced reactivity of retinal age pigment. Journal of Biological Chemistry 1995; 270(32):18825-18830.
“. . . blue light induces apoptosis in human fetal RPE cells.” E.M. Gasyna, K.A. Rezaei, W.F. Mieler, and K.A. Rezai. Blue light induces apoptosis in human fetal retinal pigment epithelium. Invest. Ophthalmol. Vis. Sci. 2005 46: E-Abstract 248.
For further scientific rationale and references regarding the potential blue light hazard, see Recommendation of protective eye wear for patients suffering from degenerative retinal diseases.(Ch. E. Remé, Laboratory of Retinal Cell Biology, University Eye Clinic, Zurich, Switzerland).
The following people are appreciated for their professional guidance and commentary in the writing of this paper. The opinions expressed herein do not necessarily reflect their positions on the issues presented.
- Roy Cole, O.D., F.A.A.O. (Director of Vision Program Development, The Jewish Guild for the Blind)
- Robert Hammer, B.Optom., M.Sc. (Petah Tikva, Israel)
- Kurt Heinmiller (MIESNA, Photometry Specialist SESCO Lighting, Inc., Fort Lauderdale FL)
- Martin A. Mainster, Ph.D., M.D., FRCOphth. (Professor and Vice Chairman/Director of Macula Service, Department of Ophthalmology, University of Kansas Medical Center)
- Lylas G. Mogk, M.D. (Chair of the American Academy of Ophthalmology Vision Rehabilitation Committee. Medical Director, Henry Ford Health System Visual Rehabilitation and Research Center, Grosse Pointe and Livonia MI)
- Donald G. Pitts, O.D., Ph.D. (Professor Emeritus of Optometry and Physiological Optics, University of Houston College of Optometry)
- Charlotte E. Remé, Ph.D. (Professor, Department of Ophthalmology, University of Zurich)
- Michael Terman, Ph.D. (Professor, Department of Psychiatry, Columbia University. President, Center for Environmental Therapeutics, New York NY.)
Reviews:
“. . . a very well written article and well understandable for lay people.” (Charlotte E. Remé, Ph.D., Prof., Dept. of Ophth., Univ. of Zurich) | |
“. . . compelling and provocative.” (Michael Terman, Ph.D., Prof., Dept. of Psychiatry, Columbia Univ., President, Center for Environmental Therapeutics) | |
“You make an excellent point regarding the toxicity of blue light and the failure of most manufacturers to recognize this and address it.”(Richard Rosen, M.D., Director of Resident Training NY Eye & Ear Infirmary) | |
“Brilliant” (Spencer Thornton, M.D., Biosyntrx, Inc.) |
References:
1 Berson DM, Dunn FA, Takao M. 2002 Phototransduction by retinal ganglion cells that set the circadian clock. Science 295: 1070-1073
2 Hattar S, Liao HW, Takao M, Berson DM, Yau KW. 2002. Melanopsin-containing retinal ganglion cells: architecture, projections, and intrinsic photosensitivity.Science 295: 1065-1070.
3 Menaker M. Circadian rhythms. Circadian photoreception. Science 2003;299:213-4.
4 Wee R, Van Gelder RN. Sleep disturbances in young subjects with visual dysfunction. Ophthalmology 2004;111:297-303.
5 Van Gelder RN. Blue light and the circadian c lock. Br J Ophthalmol 2004;88:1353.
6 Sparrow JR, Zhou J, Ben-Shabat S, et al. Involvement of oxidative mechanisms in blue-light-induced damage to A2E-laden RPE. Invest Ophthalmol Vis Sci 2002;43:1222–7.
7 Boulton M, Rozanowska M, Rozanowski B. Retinal photodamage. J Photochem Photobiol B 2001;64:144–61.
8 Rozanowska M, Jarvis-Evans J, Korytowski W, et al. Blue light-induced reactivity of retinal age pigment. In vitro generation of oxygen-reactive species. J Biol Chem 1995;270:18825–30.
9 Schutt F, Davies S, Kopitz J, et al. Photodamage to human RPE cells by A2-E, a retinoid component of lipofuscin. Invest Ophthalmol Vis Sci 2000;41:2303–8.
10 Suter M, Remé C, Grimm C, et al. Age-related macular degeneration. The lipofusion component N-retinyl-N-retinylidene ethanolamine detaches proapoptotic proteins from mitochondria and induces apoptosis in mammalian retinal pigment epithelial cells. J Biol Chem 2000;275:39625–30.
11 Sparrow JR, Nakanishi K, Parish CA. The lipofuscin fluorophore A2E mediates blue light-induced damage to retinal pigmented epithelial cells. Invest Ophthalmol Vis Sci 2000;41:1981–9.
12 Sparrow JR, Cai B. Blue light-induced apoptosis of A2E-containing RPE: involvement of caspase-3 and protection by Bcl-2. Invest Ophthalmol Vis Sci 2001;42:1356–62.< BR>
13 Rozanowska M, Korytowski W, Rozanowski B, et al. Photoreactivity of aged human RPE melanosomes: a comparison with lipofuscin. Invest Ophthalmol Vis Sci 2002;43:2088–96.
14 Sparrow JR, Zhou J, Cai B. DNA is a target of the photodynamic effects elicited in A2E-laden RPE by blue-light illumination. Invest Ophthalmol Vis Sci 2003;44:2245–51.
15 Sparrow JR. Therapy for macular degeneration: insights from acne. Proc Natl Acad Sci USA 2003;100:4353–4.
16 Rozanowska et al Blue light-induced singlet oxygen generation by retinal lipofuscin in non-polar media. Free Radic Biol Med. 1998 May;24(7-8):1107-12.
17 Rozanowska M et al. Blue light-induced reactivity of retinal age pigment. In vitro generation of oxygen-reactive species. J Biol Chem. 1995 Aug 11; 270(32): 18825-30.
18 Pawlak et al.Action spectra for the photoconsumption of oxygen by human ocular lipofuscin and lipofuscin extracts. Arch Biochem Biophys. 2002 Jul 1;403(1):59-62.
19 Verma L, Venkatesh P, Tewari H. Phototoxic retinopathy. Ophthalmology Clinics of North America. 2001;14(4): 601-609.
20 American Conference of Governmental Industrial Hygienists. Threshold limit values for chemical substances physical agents: biological exposure indices. Cincinnati: ACGIH, 1997.
21 International Commission on Non-Ionizing Radiation Protection. Guidelines on limits of exposure to broad-band incoherent optical radiation (0.38 to 3
microM). Health Phys 1997;73:539–54.
22 Sliney DH, Bitran M. The ACGIH action spectra for hazard assessment: The TLV’s. In: Matthes R, Sliney DH, ed. Measurements of optical radiation hazards. Oberschleissheim, Germany: International Commission on Non-Ionizing Radiation Protection (ICNIRP 6/98) and CIE (x016- 1998), 1998:241–29.
23 Kremers JJ, van Norren D. Two classes of photochemical damage of the retina. Lasers Light Ophthalmol 1988;2:41–52.
24 Sliney DH, Wolbarsht ML. Safety with lasers and other optical sources: a comprehensive handbook. New York: Plenum Press, 1980.
25 Kremers JJ, van Norren D. Retinal damage in macaque after white light exposures lasting ten minutes to twelve hours. Invest Ophthalmol Vis Sci 1989;30:1032–40.
26 Van Norren D, Schellekens P. Blue light hazard in rat. Vis Res 1990;30:1517–20.
27 Rapp LM, Smith SC. Morphologic comparisons between rhodopsinmediated and short-wavelength classes of retinal light damage. Invest Ophthalmol Vis Sci 1992;33:3367–77.
28 American Conference of Governmental Industrial Hygienists. Threshold limit values for chemical substances physical agents: biological exposure indices. Cincinnati: ACGIH, 1997.
29 International Commission on Non-Ionizing Radiation Protection. Guidelin es on limits of exposure to broad-band incoherent optical radiation (0.38 to 3 microM). Health Phys 1997;73:539–54.
30 Williams TP, Howell WL. Action spectrum of retinal light-damage in albino rats. Invest Ophthalmol Vis Sci 1983;24:285–7.
31 Pautler EL, Morita M, Beezley D. Hemoprotein(s) mediate blue light damage in the retinal pigment epithelium. Photochem Photobiol 1990;51:599–605.
32 Saari JC, Garwin GG, Van Hooser JP, et al. Reduction of all-trans-retinal limits regeneration of visual pigment in mice. Vis Res 1998;38:1325–33.
33 Grimm C, Remé CE, Rol PO, et al. Blue Light&# 146; s effects on rhodopsin: photoreversal of bleaching in living rat eyes. Invest Ophthalmol Vis Sci 2000;41:3984–90.< BR>
34 Grimm C, Wenzel A, Williams T, et al. Rhodopsin-mediated blue-light damage to the rat retina: effect of photoreversal of bleaching. Inve st Opht halmo l Vis Sci 2001;42:497–505.
35 Mainster MA. Light and macular degeneration: a biophysical and clinical perspective. Eye 1987;1(Pt 2):304–10.
36 Feeney L, Berman ER. Oxygen toxicity membrane damage by free radicals. Invest Ophthalmol 1976;15:789–92.
37 Snodderly DM. Evidence for protection against age-related macular degeneration by carotenoids and antioxidant vitamins. Am J Clin Nutr 1995;62(Suppl):1448–61.
38 Kennedy CJ, Rakoczy PE, Constable IJ. Lipofuscin of the retinal pigment epithelium: a review. Eye 1995;9(Pt 6):763–71.
39 Remé C, Reinboth J, Clausen M, et al. Light damage revisited: converging evidence, diverging views? Graefes Arch Clin Exp Ophthalmol 1996;234:2–11.
40 Beatty S, Koh H, Phil M, et al. The role of oxidative stress in the pathogenesis of age-related macular degeneration. Surv Ophthalmol 2000;45:115–34.
41 Robison WG, Kuwabara T, Bieri JG. The roles of vitamin E and unsaturated fatty acids in the visual process. Retina 1982;2:263–81.
42 Marshall J. The aging retina: physiology or pathology. Eye 1987;1(Pt 2):282–95.
43 Mainster MA. Light and macular degeneration: a biophysical and clinical perspective. Eye 1987;1(Pt 2):304–10.
44 Hunyor AB. Solar retinopathy: its significance for the aging eye and the younger pseudophakic patient. Aust N Z J Ophthalmol 1987;15:371–5.
45 Roberts JE. Ocular phototoxicity. J Photochem Photobiol B 2001;64:136–43.
46 Bernstein PS, Zhao DY, W int ch SW, et al . Resonance Raman measurement of macular carotenoids in normal subjects and in age-related macular de gener ation patients. Ophthalmology 2002;109:1780–7.
47 Mainster MA. Solar retinitis, photic maculopathy and the pseudophakic eye. J Am Intraocul Implant Soc 1978;4:84–6.
48 Ts’o MO, La Piana FG, Appleton B. The human fovea after sungazing. Trans Am Acad Ophthalmol Otolaryngol 1974;78:OP–677.
49 Marshall J. aging changes in human cones. Acta XXIII Concilium Ophthalmologicum (Kyoto) 1978;1:375–8.
50 Borges J, Li ZY, Tso MO. Effects of repeated photic exposures on the monkey macula. Arch Ophthalmol 1990;108:727–33.
51 Liu IY, White L, LaCroix AZ. The association of age-related macular degeneration and lens opacities in the aged. Am J Public Health 1989;79:765–9.
52 Taylor HR, Munoz B, West S, et al. Visible light and risk of age-related macular degeneration. Trans Am Ophthalmol Soc 1990;88:163–73, discussion 173–8.
53 Taylor HR, West S, Munoz B, et al. The long-term effects of visible light on the eye. Arch Ophthalmol 1992;110:99–104.
54 Cruickshanks KJ, Klein R, Klein BE. Sunlight and age-related macular degeneration. The Beaver Dam Eye Study. Arch Ophthalmol 1993;111:514–8.
55 Darzins P, Mitchell P, Heller RF. Sun exposure and age-related macular degeneration. An Australian case-control study. Ophthalmology 1997;104:770–6.
56 Cruickshanks KJ, Klein R, Klein BE, et al. Sunlight and the 5-year incidence of early age-related maculopathy: the Beaver Dam Eye Study. Arch Ophthalmol 2001;119:246–50.
57 Delcourt C, Carriere I, Ponton-Sanchez A, et al. Light exposure and the risk of age-related macular degeneration: the Pathologies Oculaires Liees a l’Age (POLA) study. Arch Ophthalmol 2001;119:1463–8.
58 McCarty CA, Mukesh BN, Fu CL, et al. Risk factors for age-related maculopathy: the Visual Impairment Project. Arch Ophthalmol 2001;119:1455–62.
59 Mellerio J. Light Effects on the Retina Principles and Practice Of Ophthalmology Chapter 116 Eds. Albert D M, Jakobiek F A, Saunders W B. Philadelphia, 1994.
60 Guidelines on Limits of Exposure to Broad-Band Incoherent Optical Radiation (0.38 to 3µm). The International Commission on Non-Ionizing Radiation Protection. Health Physics Vol. 73, No 3, pp 539-554, 1997.
61 Jackson GR, Owsley C, Cordle EP, et al. Aging and scotopic sensitivity. Vi Res 1998;38:3655–62.
62 Jackson GR, Owsley C, McGwin G Jr. Aging and dark adaptation. Vis Res 1999;39:3975–82.
63 CVRL Color & Vision database at the Institute of Ophthalmology in London. Updated: November 20th, 2003.
64 Grimm C, et al. Rhodopsin-Mediated Blue-Light Dam age to the rat Retina: Effect of Photoreversal of Bleaching. Invest Ophthalmol Vis Sci 2001 Feb;42(2):497-50.
65 Wihlmark U, Wrigstad A, Roberg K, Nilsson S, Brunk U. Lipofuscin Accumulation in Cultured Retinal Pigment Epithelial Cells Causes Enhanced Sensitivity to Blue Light Irradiation. Free Radical Biol. Med. (1997) 22, 1229-1234.
66 Kremers JJ, van Norren D. Two classes of photochemical damage of the retina. Lasers Light Ophthalmol 1988;2:41-52.
67 Kremers JJ, van Norren D. Retinal damage in macaque after white light exposures lasting ten minutes to twelve hours. Invest Ophthalmol Vis Sci 1989;30:1032-40.
68 Ham WT, Jr., Mueller HA, Sliney DH. Retinal sensitivity to damage from short wavelength ligh t. Nature 1976;260:153-5.
69 Ham WT, Jr., Ruffolo JJ, Jr., Mueller HA, Guerry D, 3rd. The nature of retinal radiation damage: dependence on waveleng th, po wer level and exposure time. Vision Res 1980;20:1105-11.
70 Threshold limit values for chemical substances physical agents: biological exposure indices. Cincinnati: American Conference of Governmental Industrial Hygienists; 1997.
71 Abbott A. Restless nights, listless days. Nature 2003;425:896-8.
72 Magnusson A, Boivin D. Seasonal affective disorder: an overview. Chronobiol Int 2003;20:189-207.
73 Hernandez-Andrés J, Romero J, Nieves JL. Color and spectral analysis of daylight in southern Europe. J. Opt. Soc. Am. Vol. 18, No. 6, pp 1325-1335, June 2001.
74 Mumford, R. Improving visual efficiency with selected lighting. Journal of Optometric Visual Development, Vol. 33, No. 3, Fall 2002.
75 Glass P. Light and the developing retina. Documenta Ophthalmologica. 1990;74(3):195-203.
76 Kini M, Leibowitz H, Colton T, Nickerson R, Ganley J, Dawber T. Prevalence of senile cataract, diabetic retinopathy, senile macular degeneration, and open-angle glaucoma in the Framingham eye study. Am J Ophthalmol. 1978;85(1):28-34.
77 Sliney D. Ocular injury due to light toxicity. International Ophthalmology Clinics. 1988;23(3) :246-250.
78 E.M. Gasyna, K.A. Rezaei, W.F. Mieler, K.A. Rezai. Blue Light Induces Apoptosis in Human Fetal Retinal Pigment Epithelium. Ophthalmology & Visual Science, University Chicago, Chicago, IL.
79 K.A. Rezai, E.M. Gasyna, K.A. Rezaei, W.F. Mieler. AcrySof Natural Filter Decreases the Blue Light Induced Apoptosis in Human Retinal Pigment Epithelium. Ophthalmology & Visual Science, University of Chicago, Chicago, IL.
80 M.Hammer, S.Richter, K.Kobuch, D.Schweitzer. Lipofuscin Accumulation in an Organotypic Perfusion Culture of Porcine Fundi Under Oxidative Stress and Blue Light Irradiation. Department of Ophthalmology, Univ. of Jena, Jena, Germany; Department of Ophthalmology, Univ. of Regensburg, Jena, Germany.
81 J.R. Sparrow, S.Kim, K.Nakanishi, Y.Itagaki. Studies of the Photooxidation of A2E and Its Precursor A2-PE. Department of Ophthalmology, Department of Chemistry, Columbia University, New York, NY.